By Steve Lundeberg
If chemistry professor May Nyman and her lab group worked in home construction, they’d be the ones building the foundation — and then moving on to the next house, leaving the floors, walls and roof to subsequent carpenters.
“We focus on the fundamentals,” Nyman says. “My group and I are molecule makers. It feels genuine and pure, and we let other people figure out how to apply our molecules. Some people do applied research very well, some start companies with their discoveries. We make inorganic molecules and want to understand how these molecules are made.”
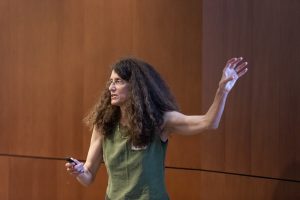
The Nyman group’s quest is understanding the structure of important elements of the periodic table like aluminum, zinc and chromium — elements that have a range of uses in electronic circuits, nuclear energy, environmental science and catalysis.
“Usually we make our molecules in water. Water is a sustainable solvent, it’s an inexpensive solvent, and it’s nontoxic,” Nyman says. “It’s a good solvent to work in if you want to make molecules that can have a useful application and be stable in an aqueous environment, like the environment we live in. Solid-state characterization is important, but understanding what is happening in solution is much more challenging and exciting.”
This year, in recognition of her achievements, Nyman received the F.A. Gilfillan Award, established in honor of the dean of the School of Science from 1939 to 1962. See Nyman’s presentation online.
Ironically, to find out what is happening in solution, a key to success for a student in Nyman’s lab is to crystallize something out of solutions formed by chemical reactions. A crystalline solid is one whose constituents — atoms, molecules and ions — are arranged in a highly ordered microscopic structure, forming a lattice that extends in every direction. They can be common like salt or beautiful like diamonds.
“Crystals hold many answers and also give rise to many questions,” Nyman says. “If you can crystallize a solid, you can analyze it and know its exact atomic arrangement and learn how the atomic arrangement leads to useful behavior. When one of my students shows me a new crystal structure, a brand-new way to link together molecules or atoms, that makes my day.”
Heavy Metal
Of particular interest to Nyman are so-called metal-oxo clusters, collections of oxygen and metal atoms larger than a molecule but smaller than a bulk solid.
Metal oxides, compounds produced when metals combine with oxygen, serve a variety of important purposes. For example, titanium dioxide is a catalyst that degrades pollutants, and aluminum oxides and iron oxides are coagulants, used as the first step in purifying drinking water.
“Metal oxides influence processes everywhere,” Nyman says. “They control the spread of contaminants in the environment. They are the touchscreen of your cellphone. The metal-oxide cluster forms are in your body storing iron and in plants controlling photosynthesis. Most of these processes are in water. Yet scientists still know so little about how these metal oxides operate in nature, or how we can make them with the absolute control needed for high-performance materials in energy applications.”
Nyman’s group works to unravel those mysteries by making metal-oxo clusters and dissolving them, characterizing how they behave in solution with changing concentrations and conditions.
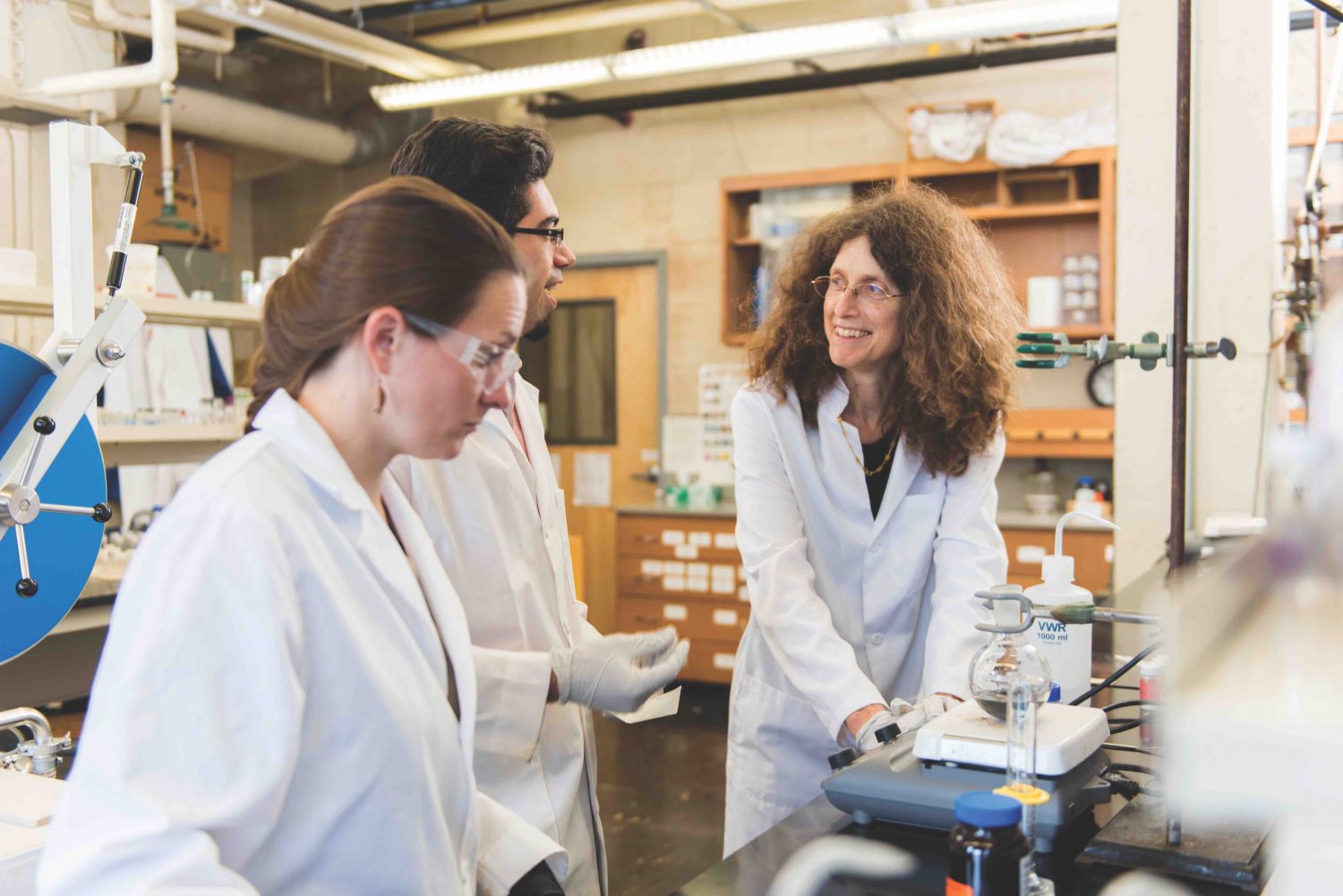
“We can use mass spectrometry and small-angle X-ray scattering to look at the atomic arrangement in solution in response to different conditions,” she says. “Understanding all of that for metal-oxo clusters across the periodic table has many implications: protein science, biological science, geosciences, building materials, understanding how contaminants transport in the environment and understanding why things are soluble or insoluble.”
Putting Chemistry to Work
Nyman joined the College of Science faculty in 2012 following nearly a decade and a half at Sandia National Laboratories in Albuquerque, New Mexico. Sandia, whose roots trace to the Manhattan Project during World War II, is one of three National Nuclear Security Administration research and development labs, with a focus on nuclear materials and nuclear weapons systems.
During her time at Sandia, Nyman focused on designing materials able to separate radioactive cesium, strontium and plutonium from nuclear waste. She led efforts to clean up contaminants old (from the Cold War) and new (from the meltdown of the Fukushima Daichii nuclear reactor in Japan).
Nyman developed a new metal-oxide sorbent — an insoluble material used to retrieve other substances through absorption or adsorption — that resulted in a 20-fold improvement in the capture of radioactive strontium. The material was 40 times more effective than other sorbents for plutonium separation and five times more effective for neptunium capture.
At Sandia, Nyman also worked on radioactive cesium removal technologies used in treating contaminated seawater at the earthquake-ravaged Fukushima power plant. The research led her to discover a new class of a type of compound known simply as a POM, or polyoxometalate: hetero polyniobates.
The latter, abbreviated to PONbs, are extremely active basic compounds that have a strong binding effect with positively charged molecules, including ones found in living organisms. The discovery opened the door for chemical warfare countermeasures, molecules that degrade nerve agents like sarin gas.
“There’s not much bandwidth for fundamental chemistry at a lab like Sandia, but this was a pretty important discovery that opened up very fundamental questions,” Nyman explains. “How anions and cations interact in solution, how synthetic materials crystallize, and how they form in nature — these are important things to know and control to make new materials.”
After almost 15 years at Sandia, Nyman sought to pivot to academia. Her top choice was Oregon State and the Center for Sustainable Materials Chemistry, with its focus on making thin-film materials from metal-oxo clusters like POMs.
She arrived in Corvallis in 2012, and in her first year, Nyman secured funding from the Department of Energy to study PONbs. She spent her first summer at OSU poring over X-ray scattering data for metal-oxo cluster chemistry, work she found so exciting that she calls that time “one of the best summers of my life.”
Nyman’s group conducts metal-oxo cluster-chemistry research all across the periodic table, including the actinides — the radioactive elements that make up the table’s bottom row. They are advancing separation technology in the nuclear fuel cycle through the use of uranyl peroxide clusters.
According to Nyman, “despite the fact that scientists made a bomb out of plutonium, there is still little known about these elements. Their electron configurations are very complicated, and how and where they fit in the big picture of the periodic table are still not well understood. My students who do uranium chemistry — because there are few scientists worldwide studying actinides — make new discoveries all time. It’s not a crowded field.”
A Lab for Students
Nyman seeks internship opportunities for her students in national labs, like the Pacific Northwest National Laboratory in Richland, Washington. This past summer, OSU Ph.D. student Ana Arteaga worked in nuclear forensics, figuring out the history of radioactive material: where it was mined, how it was processed, and where and how it was stored.
Arteaga’s work at PNNL centers on mysterious cubes of uranium thought to possibly be among those once used by the Nazis in a failed attempt to make their own atomic bomb during World War II. Many of the cubes went missing after the war. According to an Aug. 30, 2019, NPR story, one of the cubes ended up at PNNL.
“May is crazy busy all the time but somehow still finds a way to make time for her students,” Arteaga says. “I can just walk in her door, ask questions about science or my personal life or career — even now when I’m at the PNNL, I just call her and talk to her. She always makes time, while also being a mom and an amazing scientist.”
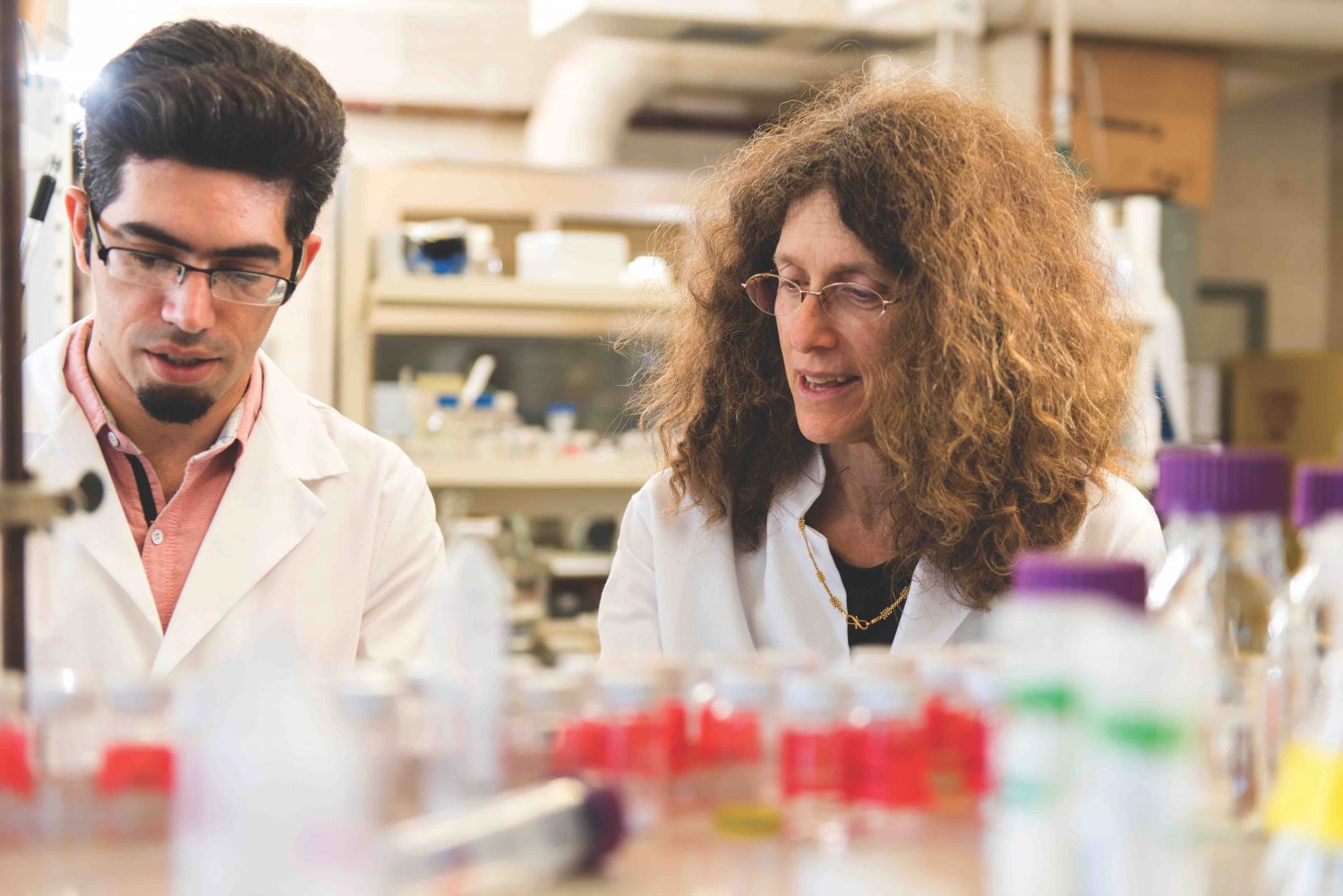
Danielle Hutchison, another Ph.D. student, marvels at how her boss keeps it all straight. “May is always working, always coming up with new ideas for experiments, new funding opportunities.”
And always supportive of her students, they attest.
“I had a baby five months ago, and May was wonderful,” Hutchison adds. “She helped me figure out maternity leave and loves my son, loves to see him, likes when I bring him into the office. I know there are many women who don’t have that kind of support in grad school.”
Morgan Olsen, another of Nyman’s 10 Ph.D. students (the lab also has one master’s student, one postdoctoral scholar and three undergraduates) says she and her lab mates “are very fortunate to have a boss like Dr. Nyman.”
“Beyond commending our hard work, she is also supportive in times of need,” Olsen says. “I have had some health issues this year, and she offered support and encouragement in multiple ways. Graduate school is an emotionally and physically draining process, and she strives to help her students in whatever way possible.”
Arteaga, the nuclear forensics intern, shares a similar experience. “I had a family emergency and had to leave school for a while. I had to take care of my uncle and my sister. May knew about the situation and consistently emailed me, asking me how I was doing. She was very supportive, even though she wasn’t even my advisor at the time. When I came back, I knew I wanted to be in her group.”
Having taught for just seven years, Nyman says her ideas on instruction are still evolving, though her mission is clear. “My job is to turn students into well-rounded, independent scientists,” she says. “There is a great need for actinide chemists in national labs, and we’re training the next generation of them.
“I would like to get more into science with applications that the general public can understand,” she adds, “but every time we go down that road, we get sidetracked by fundamental science and understanding how chemistry works in solutions, especially metal-oxo clusters. It’s what we’re good at, and we can’t get away from it.”